Cytokine Therapy
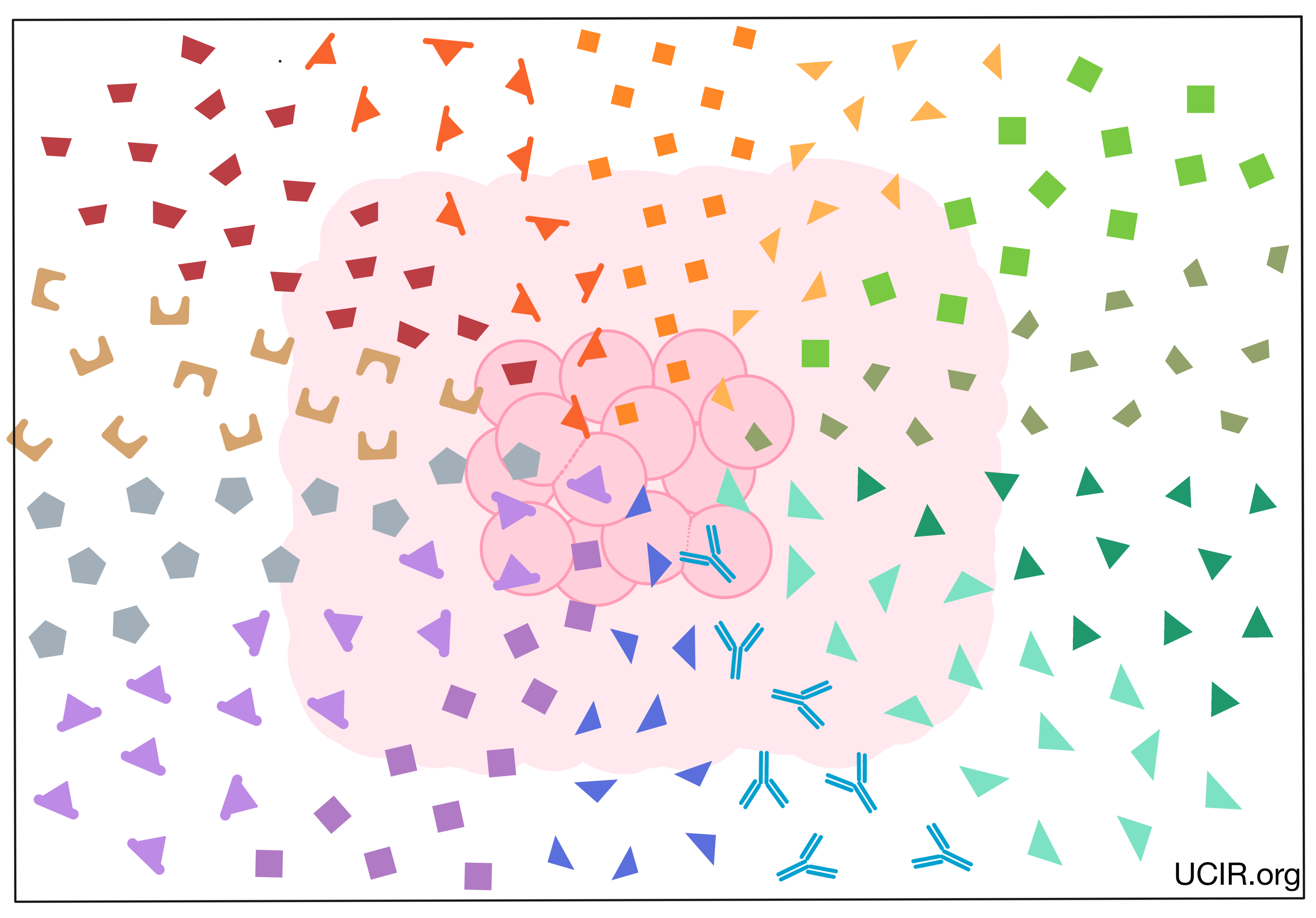
Background
The immune system is the human body’s natural defense system. It is made up of a complex network of organs, cells, and signals that can be found throughout the body. As a whole, the immune system’s role is to protect the body by recognizing anything that does not belong, including harmful bacteria, viruses, dead or damaged cells, and cancer.
The immune system has evolved as an overlapping and interconnected two-armed system. The first arm, known as the innate immune response, responds quickly (within hours) to any potential threat, while the second arm, known as the adaptive immune response, develops more slowly (over several days), but is more powerful and more specific to whatever the particular threat may be.
Central to this highly evolved and complex two-armed immune process is effective communication between immune cells both within and between arms. The communication allows immune cells throughout the body to work together to initiate a response, recruit the right immune cells to the scene, control the strength of the immune response, shut down the response once the threat has been eliminated, and generate long-term immune memory that can protect against similar threats in the future. While some communication between immune cells occurs when the cells come into direct contact, a large part of their communication occurs through the sending and receiving of small signal molecules called cytokines.
What are cytokines and chemokines?
Cytokines are small protein molecules that are released by immune cells in order to send signals to other cells, typically in response to a threat or activating event, such as an infection. Cytokines can spread locally within a tissue or can travel long distances through blood or lymph, sending signals throughout the body. Each cytokine has a corresponding receptor or receptors that can receive the signals. When a cytokine reaches a cell that has the right receptor to receive the signal, it binds to the receptor, and influences that cell’s function, activity, or movement. For example, different cytokines may stimulate immune cells to mature and develop cell-killing functions, multiply in number, or move throughout the body. Other cytokines may dampen the immune response in order to limit tissue damage, or end the immune response once the target infection or threat has been destroyed. Further, one cytokine can sometimes act on many different cell types, causing the same or sometimes different effects in different cells or locations. Overall there are nearly 100 known cytokines, some of which may have overlapping functions, may influence one another, or may have different effects in combination.
The cytokine family can be divided into two subgroups: cytokines and chemokines.
- Cytokines: molecules that send various signals and messages that affect the functions and growth of immune cells. There are currently more than thirty known members of this group.
- Chemokines: molecules that direct the movement and positioning of immune cells. Chemokines may also direct fluid and other immune molecules. There are currently more than fifty known members of this group.
Cytokines in cancer immunotherapy
Given their abilities to affect the numbers and functions of immune cells, cytokines have long been utilized in cancer immunotherapy, and could be considered the earliest form of cancer immunotherapy. Cytokines can have multiple roles in the immune system, serving as both activators and dampeners of immune responses. When it comes to immune activators, cytokines produced in a laboratory can be administered to a patient to enhance immune responses against cancer. Alternatively, blocking the activity of cytokines that act as immune dampeners can also help sustain or improve anti-cancer immune responses. Blocking the activity of a cytokine can be achieved by targeting either the cytokine or its receptor. Cytokine therapies may also be used in combination with other cancer treatments to enhance their effects.
Examples of cytokine-based therapies
Cytokine-enhancing therapies:
Interferon alpha (IFNα)
Interleukin 2 (IL-2)
Interleukin 15 (IL-15)
Interleukin 12 (IL-12)
Interleukin 10 (IL-10)
Granulocyte-macrophage colony stimulating factor (GM-CSF)
Cytokine-blocking therapies:
Blocking tumor necrosis factor alpha (TNFα)
Blocking transforming growth factor beta (TGFβ)
Blocking interleukin 6 (IL-6)
Interferon alpha (IFNα)
IFNα is a family of closely related proteins, which are part of a larger family of proteins known as Type 1 interferons. Interferons were first identified by their ability to “interfere” with viral infections. In early studies, INFα was found to enhance anti-cancer immune functions, promote cancer cell death in some cancers, and suppress the development of blood vessels in some solid tumors.
Different members of the IFNα family (interferon alpha-2a and interferon alpha-2b) have been used to treat several types of cancer and have provided benefits in some patients. However, there is significant toxicity associated with IFNα therapy, including fever, fatigue, headache, stomach and intestinal symptoms, muscle pain, reduction in several blood cell types, and psychiatric issues such as depression. IFNα has been approved in the US under the brand names IntronA and Sylatron, for use in hairy cell leukemia, melanoma, follicular lymphoma, and AIDS-related Kaposi's sarcoma. In recent years, newer, less toxic therapies, and the use of combination therapies that block immune checkpoints have replaced IFNα therapy and pushed it to a second-line option.
Even though newer therapies have reduced the use of IFNα as a cancer therapeutic, its history of effectiveness proves its potential, and many new strategies are being tested to reduce the toxicities associated with its use. These include combining IFNα with cell-based therapies, cancer vaccines, and checkpoint inhibitors.
Interleukin 2 (IL-2)
IL-2 is a cytokine that promotes the multiplication and activation of cytotoxic T cells – immune cells that can kill infected or cancerous cells. As with many cytokines, though, its functions are complex, and IL-2 also plays a role in shutting down antitumor activity by promoting multiplication of “regulatory” T cells, which suppress the activity of cytotoxic T cells.
Treatment with high doses of IL-2 (Proleukin) is currently approved for use in advanced kidney cancer and melanoma, though it is limited by its significant toxicity.
In addition to being used as a direct therapy, IL-2 also has important uses in the laboratory, as IL-2 is used to grow and activate anti-cancer T cells that have been taken from a cancer patient’s blood or tumor for use as a T cell therapy. After treatment with IL-2, these cells are then given back to the patient along with more IL-2 to further activate and expand their number in the patient; clinical trials using this strategy show that it can be incredibly effective.
Given its history of efficacy, many second-generation IL-2-based strategies are being tested to improve efficacy while reducing toxicity. Some strategies include chemically linking IL-2 to a compound or antibody that delivers it to the tumor, thus making it possible to reduce the dose of IL-2 and the toxic effects it causes in the rest of the body. Other strategies include genetically engineering IL-2 to limit the cell types it binds to so that it can activate cells that have cancer-fighting functions, but not those that cause toxic side effects.
Interleukin 15 (IL-15)
IL-15 is cytokine that interacts with the IL-15 receptor on the surface of T cells and NK cells, and signals them to increase in number and develop cancer cell-killing activities. Importantly, IL-15 does not stimulate the activation of regulatory or “suppressor” T cells that work to shut down the anti-cancer immune response.
IL-15 was found to be effective as a treatment for cancer in several animal models, and the effect depended on tumor cell killing by NK cells. In a phase I clinical trial that used IL-15 alone to treat adults with advanced melanoma and kidney cancer, there were hints of activity with limited toxicity. The IL-15 treatment caused significant changes in the distribution of NK cells in the body, suggesting that these cells played a role in the responses. In another study, activated NK cells were transferred into patients with acute myelogenous leukemia, and the addition of IL-15 induced significant clinical responses.
The receptor for IL-15 is made up of two molecules. IL-15 first binds to one half of the receptor, forming a unit that can bind to the second half of the receptor to activate NK cells and cytotoxic T cells. To improve the efficacy of IL-15 as a therapy, scientists have engineered proteins where IL-15 is already linked to the first half of the IL-15 receptor, making it easier to complete the binding process. Two forms of these engineered IL-15 proteins are currently in clinical trials in combination with checkpoint inhibitors. Thus far, the drug has been shown to be well tolerated and effective at increasing the numbers and stimulating the activation of NK cells and cytotoxic T cells, without increasing suppressive T cells. Responses to treatment were observed in 19% of patients, including one patient who experienced complete remission that lasted for 7 months. Further studies are being planned.
NK cells recognize and kill cells that are coated in antibodies – a process called antibody-dependent cell-mediated cytotoxicity (ADCC). Thus, based on the observations that IL-15 acts primarily on NK cells, additional clinical studies are planned to treat patients with IL-15 in combination with antibodies that bind to patients’ cancer cells.
Interleukin 12 (IL-12)
IL-12 is a cytokine that is made primarily by cells of the innate immune system in response to “danger signals”, like the molecules released when invading bacteria are broken down. IL-12 is critical in driving the development of helper T cells, immune cells that promote the development of cancer-killing cytotoxic T cells. Helper T cells are critical in anti-cancer responses. Additional studies have shown that IL-12 can also limit cancer growth by blocking the formation of a tumor environment that supports the tumor.
Administration of IL-12 to patients with cancer has proved to be too toxic for direct use. However, the potent anti-cancer activity of IL-12 has kept interest in it high, and several strategies are being explored to bring its potential to fruition. Strategies to reduce the toxicity of IL-12 revolve around delivering it locally to tumor sites, or targeting it so that it preferentially accumulates at tumor sites. One approach involves genetically engineering oncolytic viruses to produce IL-12. When delivered to a patient, these viruses would preferentially infect tumor cells and induce local production of IL-12 to activate immune cells in the tumor. Another similar strategy involves injecting DNA that codes for IL-12 into tumors, so that local cells take up the DNA and begin to produce IL-12 in the tumor. Another strategy is to link IL-12 to an antibody to target it to the tumor site (or keep it there if it is injected directly into the tumor). All of these options can be in combination with checkpoint blockade. Finally, T cell therapies can also benefit from IL-12. One strategy is to genetically engineer CAR T cells to produce IL-12, which helps to keep the CAR T cells alive and active in the tumor.
Interleukin 10 (IL-10)
IL-10 is a complex cytokine with both immune-activating and immune-suppressing activities, and has a role in balancing the immune response. Recent studies have shown that its activity can vary depending on its environment. In a cancer setting, IL-10 is crucial in preventing the death of cytotoxic T cells – immune cells that can kill cancer cells. The potential benefit of IL-10 was explored in a clinical study in several types of cancer. It was well tolerated, and tumor shrinkage was observed in several patients. In a subsequent study, which combined IL-10 with checkpoint inhibitors, 50% of patients achieved significant tumor shrinkage and 9% saw their cancer entirely disappear.
Granulocyte-macrophage colony stimulating factor (GM-CSF)
GM-CSF is a cytokine that supports the development and activation of innate immune cells, including granulocytes, macrophages, and some types of dendritic cells. These cells collect debris from damaged or dying cells, and present this debris to T cells (immune cells that can kill cancer cells) as evidence of a potential threat. The T cells that recognize the debris become activated, multiply, and move to attack the threat. GM-CSF can also directly enhance the functions and activities of T cells.
GM-CSF has been used to enhance the effectiveness of vaccines against tumor targets. For example, GM-CSF is a component of the FDA-approved drug Imlygic (talimogene laherparepvec), which can be used to treat melanoma.
Blocking tumor necrosis factor alpha (TNFα)
TNFα is a cytokine that acts as a major driver of inflammation, and while it can serve as an immune activator early in an immune response, recent studies have shown that long-term exposure to TNFα can actually suppress T cell responses and promote tumor growth. Thus, clinical studies are investigating whether blocking TNFα using a clinically approved antibody could improve the efficacy of immunotherapies, such as checkpoint blockade therapies.
Blocking transforming growth factor beta (TGFβ)
TGFβ is a cytokine with many functions. In the initial phases of tumor development, TGFβ can block the growth of cells that have become cancerous. However, in late phases of tumor development, it can act to help draw in blood vessels, which support the tumor and provide it with nutrients. TGFβ can also recruit immune cells that dampen the anti-cancer immune response. Drugs that block TGFβ have been developed to limit these effects, but have not shown strong results when delivered alone. However, they appear to have activity in combination with other immunotherapies, such as checkpoint inhibitors. Thus several clinical trials are in progress to test the safety and anti-cancer effects of blocking TGFβ in combination with checkpoint inhibitors.
Blocking IL-6
IL-6 is a cytokine that can be produced by many different types of immune cells, including T cells, B cells, and monocytes. When it is produced at normal levels, IL-6 plays a role in promoting inflammation and immunity, but at higher-than-normal levels, it can cause lymphocytes (particularly B cells) to multiply too rapidly and accumulate within lymph nodes, leading to the enlargement of the lymph nodes and other symptoms of disease. Blocking IL-6 prevents it from binding to its receptor (which may be floating freely in body fluids or found on the surface of immune cells). This prevents lymphocytes from multiplying out of control, thus controlling the cancer and its symptoms. An antibody drug called Sylvant (siltuximab) is approved for use in patients with Multicentric Castleman’s disease. Sylvant binds to IL-6 and prevents it from binding to its intended receptor.
Chemokines in cancer immunotherapy
Chemokines are one of the two subgroups of cytokines. Chemokines direct cell movement, and play an important role in organizing the distribution of immune cells and structural cells throughout the body. For example, chemokines can cause immune cells to move from the blood to sites of infection or cancer, or from the sites of infection or cancer to immune organs such as lymph nodes, where other immune cells can become activated to initiate a targeted attack. Chemokines also play a role in recruiting cells that modify or repair tissue. Like cytokines, chemokines work through specific receptors. Often, a particular receptor can bind to multiple chemokines, and a particular chemokine can bind to multiple receptors, leading to some overlapping functions.
Examples of chemokine-based therapies
CCR4
CCR1
CCL2 and its receptor CCR2
CCR7
CXCR2
CXCR4
CCR4
CCR4 is a chemokine receptor that is present at high levels on the surface of many leukemias and lymphomas, as well as on a class of immune cells called regulatory T cells, which suppress anti-cancer immune responses. An antibody that specifically binds the chemokine receptor CCR4 can eliminate both cancer cells and regulatory T cells. In a clinical trial, these antibodies depleted cancer cells, enhanced immune responses to cancer, and improved the effects of a checkpoint blockade therapy.
An antibody drug called Poteligeo (mogamulizumab), which binds to CCR4 on cancerous T cells, is approved for use in patients with Mycosis fungoides or Sézary syndrome. Poteligeo works to kill cancer cells through antibody-dependent cell-mediated cytotoxicity (ADCC). When bound to CCR4 on the surface of cancerous or normal T cells, Poteligeo can also attract and bind immune cells like NK cells, allowing Poteligeo to act as a bridge between the target cell and the immune cell. The immune cell then releases molecules that can kill the cell that Poteligeo is bound to.
CCR1
CCR1 is a chemokine receptor that is present on many types of immune cells. Blockade of CCR1 in several mouse tumor models reduced tumor growth and metastasis (the spread of tumors to new, distant sites). In addition, when a CCR1-blocking agent was used in combination with a checkpoint inhibitor, the effect of both therapies together was able to limit cancer growth in a mouse model of breast cancer. Across these studies, blocking CCR1 seemed to prevent tumors from recruiting myeloid cells, which are thought to support tumors and promote metastasis – the spread of cancer to distant sites.
CCL2 and its receptor CCR2
Blockade of the chemokine CCL2 and/or its receptor CCR2 on immune cells has anti-cancer activity in many mouse models of cancer. It is thought to act by reducing the recruitment of a type of immune cell called a monocyte, which promotes tumor development and metastasis (the spread of tumors to new, distant sites). Clinical studies of CCR2 inhibitors in combination with other therapies have been encouraging, but inconclusive. Nevertheless, the data suggest that targeting CCL2/CCR2 has potential efficacy, and studies are ongoing.
CCR7
CCR7 is present in high amounts on the surfaces of many tumors, and it appears to help tumors grow and metastaze (spread to new, distant sites). In mouse models of cancer, reducing CCR7 in tumor cells resulted in fewer metastases in colon cancer and inhibited growth of prostate cancer. Studies have also shown that antibodies to CCR7 could inhibit a T cell leukemia from forming metastases in the brain.
CXCR2
CXCR2 is present on the surfaces of many cancer cells and appears to aid cancer cells in resisting chemotherapy. In several mouse models of cancer, deleting the gene for CXCR2 so that it was no longer present in the cancer cells led to better responses to chemotherapy. Blocking CXCR2, thereby preventing it from sending signals to the cancer cell, had a similar effect. Additionally, blocking CXCR2 prevented the tumor from recruiting immune cells called myeloid cells, which suppress immune activity. With fewer myeloid cells in the tumor, immune responses against the cancer were enhanced. A clinical trial using a CXCR2 inhibitor to treat pancreatic cancer is currently underway, as is a study using a CXCR2 inhibitor in combination with paclitaxel in breast cancer.
CXCR4
CXCR4 is present on the surfaces of many types of cancer cells, including breast cancer, ovarian cancer, melanoma, and prostate cancer. The presence of CXCR4 on cancer cells is often associated with greater likelihood that they will metastasize (spread to new, distant sites). A drug, LY2510924, has been developed to block molecules from binding to CXCR4, preventing it from promoting growth or metastasis in cancer cells. Clinical studies testing the efficacy of LY2510924 alone and in combination with other anti-cancer drugs have shown some benefits and tolerable side effects. Additional studies continue to explore the potential benefit of blocking CXCR4.
Ongoing research
The activities of cytokines and chemokines make them potential targets for therapies that could help enhance an immune response to cancer or suppress the systems that support tumor growth. Several cytokine therapies have provided benefits to patients with cancer. However, these molecules are highly diverse and often serve a variety of complex and overlapping, or even contradicting functions.
Receptors for cytokines and chemokines can be present on immune cells, cancer cells, and other normal tissues, so therapies intended to support immune cells could potentially promote the growth of tumors, support the migration of tumor cells, or affect cells elsewhere in the body, which could cause significant symptoms, side effects, and damage. In many cases, the high doses of cytokines that would be required to induce the desired effect at the tumor site are too high for the rest of the body to tolerate.
Current efforts to improve cytokine-based immunotherapies are focused on ways to enhance the specificity and effects of cytokines, while limiting their toxicities. This includes modifying or engineering cytokines, delivering cytokines directly to tumor sites, finding ways to guide cytokines to tumor sites, keeping the cytokines inactive until they gets to the tumor site, and developing antibodies or drugs that more specifically and effectively bind to cytokines and receptors to enhance or block their activity.
Importantly, cytokines are also being incorporated into other types of therapies to enhance their antitumor effects. For example, cytokines may be given in combination with checkpoint blockades, T cell therapies or other cell-based therapies. Additionally, some researchers are incorporating cytokines into oncolytic viruses or CAR T cells, allowing them to produce useful cytokines within tumor sites. Cytokines are also used in laboratories to develop and support cells that will later be used for cell therapies.
Further information
Scientific references:
- Cytokines in the treatment of cancer (Review article) Conlon KC, Milijkovic MD, Waldmann TA. Journal of Interferon & Cytokine Research (2019)
- Cytokines in clinical cancer immunotherapy (Review article) Berraondo P, Sanmamed MF et al. British Journal of Cancer (2018)
- Cytokines in Cancer pathogenesis and cancer therapy (Review article) Dranoff G. Nature Reviews Cancer (2004)
- Chemokines and Chemokine Receptors: New Targets for Cancer Immunotherapy (Review article) Poeta VM, Massara M, Capucetti A, Bonecchi R. Frontiers in Immunology (2019)